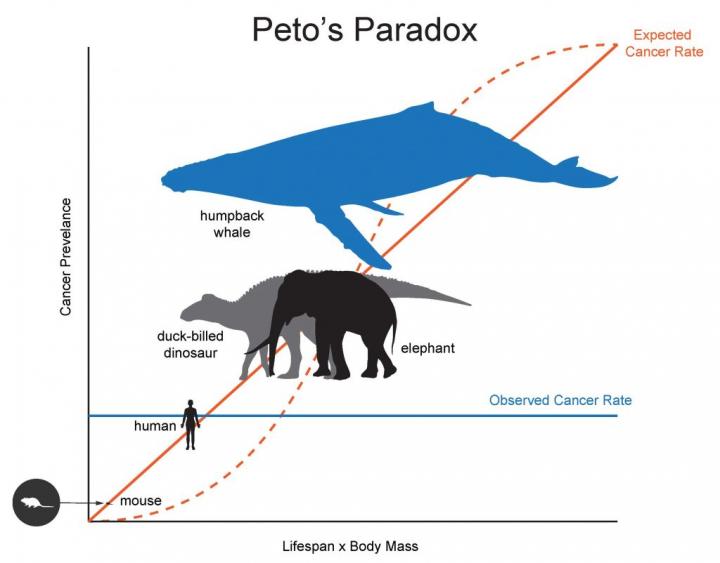
What is Peto’s Paradox?
Peto’s Paradox is named after epidemiologist Richard Peto, who noted the relationship between time and cancer when he was studying how tumors form in mice. Peto observed that the probability of cancer progression was related to the duration of exposure to the carcinogen benzpyrene. He later added body mass to the equation, when he wondered why humans both contain 1000 times more cells and live 30 times longer than mice, yet the two species do not suffer incredibly different probabilities of developing cancer. Further, cancer was not a major cause of mortality for large and long-lived wild animals, despite the increased theoretical risks. How can this be?
Why is it a paradox?
In a multicellular organism, cells must go through a cell cycle that includes growth and division. Every time a human cell divides, it must copy its six billion base pairs of DNA, and it inevitably makes some mistakes. These mistakes are called somatic mutations. Some somatic mutations may occur in genetic pathways that control cell proliferation, DNA repair, apoptosis, telomere erosion, and growth of new blood vessels, disrupting the normal checks on carcinogenesis. If every cell division carries a certain chance that a cancer-causing somatic mutation could occur, then the risk of developing cancer should be a function of the number of cell divisions in an organism’s lifetime. Therefore, large bodied and long-lived organisms should face a higher lifetime risk of cancer simply due to the fact that their bodies contain more cells and will undergo more cell divisions over the course of their lifespan. However, a 2015 study that compared cancer incidence from zoo necropsy data for 36 mammals found that a higher risk of cancer does not correlate with increased body mass or lifespan. In fact, the evidence suggested that larger long-lived mammals actually get less cancer. This has profound implications for our understanding of how nature has solved the cancer problem over the course of evolution.
How can you translate a solution in some other species to prevent cancer in humans?
Ideally, comparative studies could highlight potential targets where the genetic mechanisms underlying cancer suppression in one species could be transferred to another, with clinical implications. For instance, it was found that genetically altering mice to overexpress a form of the TP53 protein conferred a cancer-suppressive phenotype; however, these mice also displayed a premature ageing phenotype. Surprisingly, another study created ‘super p53’ mice which contained extra copies of the TP53 gene—similar to the elephant genome—under their normal promoters, and these mice revealed an enhanced DNA damage response and cancer suppression without the ageing effect. Work is now underway to develop medicines based on the TP53 pathway. While the search for solutions to Peto’s Paradox across a diversity of species is still in progress, it will no doubt require substantial effort to translate recent discoveries into effective therapies for humans.
But wait, why haven’t all animals evolved extra tumor suppression mechanisms?
Cancer is a potential problem for all multicellular life and there is no expectation that a species should be completely cancer free; in fact, elephants still get cancer—about 5% of deaths in zoos according to one study. Cancer has also been found in whales. There are a few potential reasons that cancer is still a problem for multicellular animals. First, cancer defense mechanisms, such as DNA repair, cell cycle control, and immune function, can be costly. There are likely energetic trade-offs between cancer suppression and other important life history components, such as reproduction and growth. Cancer is a disease of ageing populations both because there is weaker selection to avoid problems after reproduction, and because it takes time to accumulate all the mutations necessary to cause a cancer. For animals that are short-lived (such as mice), it doesn’t make much sense to invest much in cancer defense mechanisms. These animals are more likely to die of other extrinsic causes (such as predators) than of cancer. Second, benefits early in life that increase an organism’s fitness may lead to disease susceptibility later in life, an evolutionary term called antagonistic pleiotropy. For example, there may be a genetic variant that allows an organism to get big fast—increasing its mating potential and decreasing the likelihood it will be killed by a predator—but this same genetic variant may also lead to cancer susceptibility as the animal ages.